Treating human disease at the genetic level, by replacing defective genes or adding new genes to enhance native functions, has been a long-standing goal, with the first attempts at gene editing dating back to the 1980s1. However, it wasn’t until 2012 that momentum in the field surged, thanks to the discovery of the CRISPR/Cas9 genome editing system. Compared to the initial wave of genome editors like zinc-finger nucleases (ZFNs) and transcription activator-like effector nucleases (TALENs), CRISPR gave researchers the unprecedented ability to target and modify DNA with exceptional precision and ease, ushering in a new era of genomic medicine (Figure 1).
In late 2023, gene-edited medicine reached a significant milestone with the FDA approval of Casgevy. Developed by Vertex Pharmaceuticals Inc. and CRISPR Therapeutics, Casgevy is a CRISPR-edited gene therapy to treat sickle cell disease (SCD) and beta thalassemia (TDT). With the field continuing to advance rapidly, innovations like base and prime editing are further refining precision gene editing and expanding its potential therapeutic applications1.
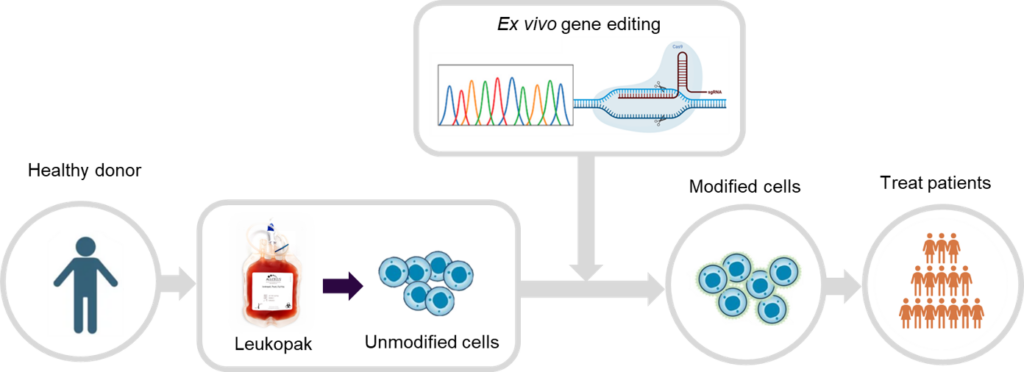
Figure 1. Ex vivo gene editing workflow.
We explore how donor-derived leukopaks support gene editing strategies to develop HSPC-based gene therapies to treat a range of diseases, as well as its role in improving the safety, efficacy, and patient accessibility of CAR-T therapies.
HSPCs and Mobilized Leukapheresis for Gene Therapy
Leading biopharma companies are developing ex vivo hematopoietic stem and progenitor cell (HSPC)-based gene therapies to treat blood-specific disorders such as primary immunodeficiencies (PIDs) and hemoglobinopathies, as well as metabolic diseases like leukocyte adhesion deficiency-I and rare conditions including Gaucher and Hunter syndrome2.
Since CD34+ cells make up only ~0.01-0.05% of non-mobilized leukapheresis collections, mobilization is commonly used to increase yield. Healthy donors receive mobilization agents to stimulate CD34+ release from bone marrow before leukapheresis3. Mobilized leukopaks provide a rich source of CD34+ HSPCs from a single donor, with yield and quality being critical for successful genetic manipulation. These factors are influenced by donor variability, mobilization regimen, and leukapheresis collection parameters.
Mobilization Regimens
FDA-approved agents granulocyte colony-stimulating factor (G-CSF) and Plerixafor are commonly used for peripheral blood mobilization. While effective on their own, dual mobilization has been shown to significantly increase HSPC yield3. However, mobilization agents like G-CSF not only mobilize stem cells, but they also stimulate granulocyte production, which increases their numbers within a mobilized leukopak4. While some granulocytes are expected, excessive contamination can compromise CD34+ cell quality and negatively impact cell viability and functionality.
Optimizing the mobilization process and collection protocols is critical to controlling granulocyte levels and ensuring high-quality, high-yield CD34+ cell collections to mitigate quality issues that could affect gene editing efficiency downstream.
Donor Variability
Additionally, donors can vary in their levels of HSPCs—some individuals naturally exhibit higher CD34+ levels or react better to mobilization agents than others, and other donor attributes such as age and BMI can impact yields. A vendor with a robust donor pool and strong donor management program is a significant advantage to researchers. The ability to identify the right donors and recall them for repeat donations at later timepoints provides a more consistent and reliable supply of CD34+ cells to support gene-editing workflows.
Download the AllCells® Mobilized Leukopak Tech Note for more detailed information on the impact of mobilization on CD34+ yield and granulocyte contamination.
Improving Gene Editing Efficiency in CAR-T Cells with High-Quality Leukopaks
CAR-T cell therapy has transformed cancer immunotherapy, but several hurdles limit its widespread adoption as a first-line treatment. Gene editing technologies are addressing these limitations by improving in vivo efficacy, reducing off-target toxicities, and enabling the development of universal CAR-T therapies to broaden patient access and reduce manufacturing costs4-6.
The efficiency of gene editing in CAR-T cells depends on the health and fitness of the starting T cell population, making leukopak quality a critical factor. For example, CRISPR/Cas9 introduces DNA double-strand breaks (DSBs) at the target locus, activating one of two endogenous repair pathways: non-homologous end joining (NHEJ) or homology-directed repair (HDR). While HDR enables precise genome modifications, it is less efficient than NHEJ and requires actively cycling cells (S and G2 phases), where homologous sister chromatids are available for repair8,9. Poor cell viability can hinder HDR efficiency and reduce the success of gene editing. Therefore, leukopaks that contain highly viable and functional cells can better support gene-editing innovations that improve CAR-T cell therapy.
Minimizing Off-Target Toxicities: Gene editing is enhancing CAR-T cell safety by preventing unintended damage to healthy cells. Strategies include optimizing CAR design for greater target specificity and engineering “safety switches” that allow controlled deactivation of CAR-T cells if adverse events occur7.
Enhancing In Vivo Efficacy: CAR-T therapy has shown limited success in solid tumors due to the immunosuppressive tumor microenvironment (TME). Gene editing can improve CAR-T cell survival, metabolism, and responsiveness to tumor signals, improving their in vivo persistence and efficacy within the TME5,6.
Engineering Universal CAR-T Therapies: Gene editing supports the development of “universal” CAR-T cells. Knock out of the native T cell receptor (TCR) and other immune-reactive genes in donor-derived T cells mitigates the potential for immune rejection and graft-versus-host disease (GvHD) in patients and enables a more cost-effective “off-the-shelf” treatment strategy8.
Conclusion
In this final post of our leukopak applications blog series, we’ve explored how the quality of donor-derived leukopaks influences the success of gene editing—whether in advancing HSPC-based gene therapies or improving CAR-T cell therapies. Along with our previous discussions on how leukopak quality affects T cell expansion, CAR-T development, and CAR-NK therapies, these insights highlight the critical role of a well-managed, reliable supply of high-quality leukopaks from donors with the right characteristics. Achieving this requires optimization and control of donor- and process-dependent variables need to preserve cell viability and functionality. As cell and gene therapies continue to advance, a reliable supply of high-quality, well-characterized leukopaks will be essential to driving innovation and delivering life-changing treatments to patients.
View the previous blogs from this series below:
Blog 1: How Leukopak Quality Impacts T-Cell Expansion and CAR-T Cell Therapy
Blog 2: Advancing CAR-NK Therapy: Why Leukopak Quality and Donor Characterization Matter
References
- Cheng A, Harikrishna JA, Redwood CS, Lit LC, Nath SK, Chua KH. Genetics Matters: Voyaging from the Past into the Future of Humanity and Sustainability. Int J Mol Sci. 2022;23(7):3976. Published 2022 Apr 2. doi:10.3390/ijms23073976
- Haltalli MLR, Wilkinson AC, Rodriguez-Fraticelli A, Porteus M. Hematopoietic stem cell gene editing and expansion: State-of-the-art technologies and recent applications. Exp Hematol. 2022;107:9-13. doi:10.1016/j.exphem.2021.12.399
- Discovery Life Sciences. Technical Note: AllCells® Mobilized Leukopaks with High CD34+ Yield and Low Granulocyte Content. November Accessed March 7, 2025. https://allcells.com/wp-content/uploads/2024/11/AllCells-Technical-Note-Mobilized-Leukopak.pdf
- Moradi V, Khodabandehloo E, Alidadi M, Omidkhoda A, Ahmadbeigi N. Progress and pitfalls of gene editing technology in CAR-T cell therapy: a state-of-the-art review. Front Oncol. 2024;14:1388475. Published 2024 Jun 7. doi:10.3389/fonc.2024.1388475
- Lei T, Wang Y, Zhang Y, et al. Leveraging CRISPR gene editing technology to optimize the efficacy, safety and accessibility of CAR T-cell therapy. Leukemia. 2024;38(12):2517-2543. doi:10.1038/s41375-024-02444-y
- Wei W, Chen ZN, Wang K. CRISPR/Cas9: A Powerful Strategy to Improve CAR-T Cell Persistence. Int J Mol Sci. 2023;24(15):12317. Published 2023 Aug 1. doi:10.3390/ijms241512317
- Lu L, Xie M, Yang B, Zhao WB, Cao J. Enhancing the safety of CAR-T cell therapy: Synthetic genetic switch for spatiotemporal control. Sci Adv. 2024;10(8): eadj6251. doi:10.1126/sciadv.adj6251
- Mishra AK, Qasim W. Genome editing approaches for universal chimeric antigen receptor T cells. EJC Paediatric Oncol. 2024;3:1001492024. doi: 1016/j.ejcped.2024.100149.
- Mikkelsen NS, Bak RO. Enrichment strategies to enhance genome editing. J Biomed Sci. 2023;30(1):51. Published 2023 Jul 1. doi:10.1186/s12929-023-00943-1